We develop and apply coarse-grained models for proteins at different levels of resolution, from detailed atomistic models to simple lattice models. Our overall goal is to discover the principles that underlie the structure, function and evolution of proteins, as well as how some molecular processes lead to disease.
Fold-switching proteins
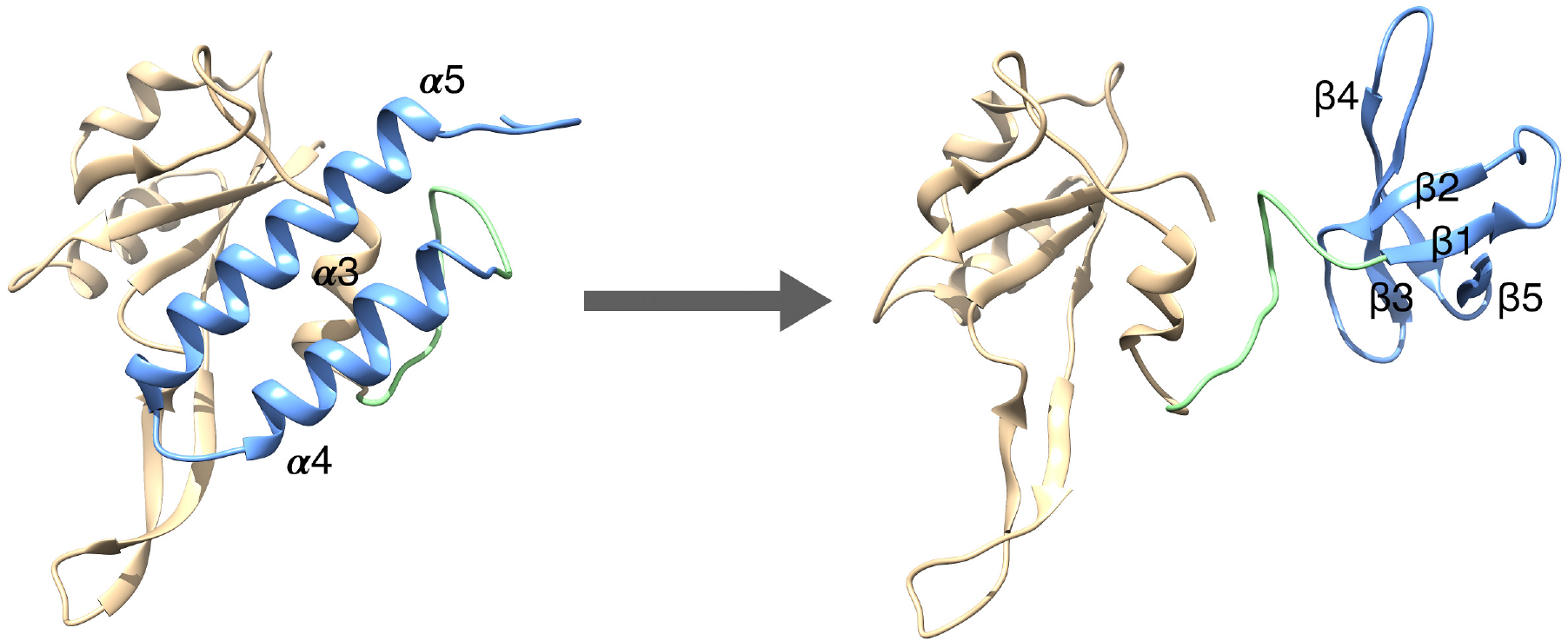
Simulating these highly specific and large-scale fold-switching events is challenging and requires new models and conformational sampling methods. We have developed a simulation strategy that is based on an all-atom model for protein folding in combination with Monte Carlo sampling techniques.
One focus of our studies is the bacterial transcription factor protein RfaH, which exhibits one of the most dramatic fold switches discovered so far. Using our simulation approach, we have probed physical properties of this protein, including structural fluctuations and mechanical properties. We are also working on understanding the mechanism that triggers the fold switch in RfaH inside bacterial cells.
Related articles:
2) Bahman Seifi, Adekunle Aina and Stefan Wallin. Structural fluctuations and mechanical stabilities of the metamorphic protein RfaH. Proteins: structure, function and bioinformatics 89 289-300 (2021)
3) Christian Holzgräfe and S. Wallin. Smooth functional transition across a mutational pathway with an abrupt protein fold switch. Biophysical Journal 107 1217-1225 (2014).
4) Robert B. Best. Bootstrapping new protein folds. Biophysical Journal 107 1040-1041 (2014). New and Notable article.
Liquid-liquid phase separation
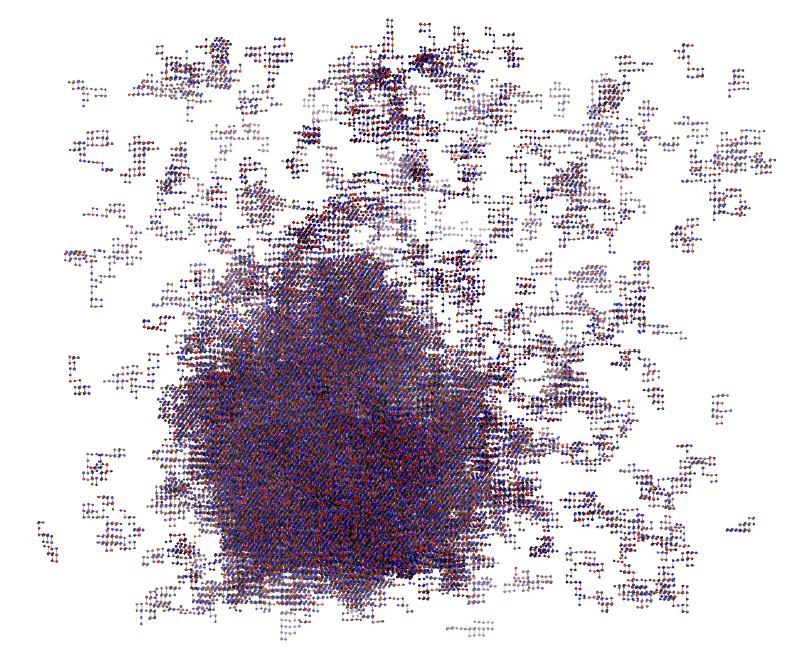
We study the properties of these liquid droplets, such as stability and concentration, and how they depend on the amino acid sequence of the disordered proteins. In particular, we have within a lattice model investigated the role of "blocks" of charged amino acids along the sequence of the disordered proteins, which are known to play an important role in the phase separation process.
Related articles:
2) Stefan Wallin. Intrinsically disordered proteins: structural and functional dynamics. Research and Reports in Biology. 8 7--16 (2017).
Other ongoing projects:
- Protein folding and macromolecular crowding.
- Coarse-graining in biomolecular simulations.
- Techniques for enhanced conformational sampling.